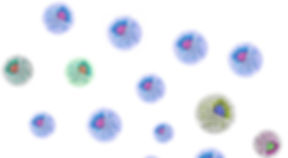
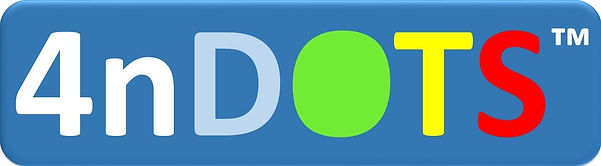
FLUORESCENT NANOPROBES
Overview
​
4nDOTS™ Fluorescent Nanoprobes are quantum-dots from semiconducting materials with diameters in the range of a few nanometers (few tens of atoms). 4nDOTS™ have unique properties, intermediate between those of bulk materials and discrete molecules that are partly the result of the unusually high surface-to-volume ratios for these particles [1-3]. The most striking feature is fluorescence, wherein the 4nDOTS™ can produce distinctive colors determined by the size of the particles.
PHORNANO offers a variety of fluorescent nanoprobes featuring bright emissions, narrow emission sprectra, high purity, and good quantum yields. They are available in aqueous medium and are applied in photonics, displays, photovoltaics, microelectronics, quantum computing, biomedical imaging, biosensors, cosmetics, among many others.
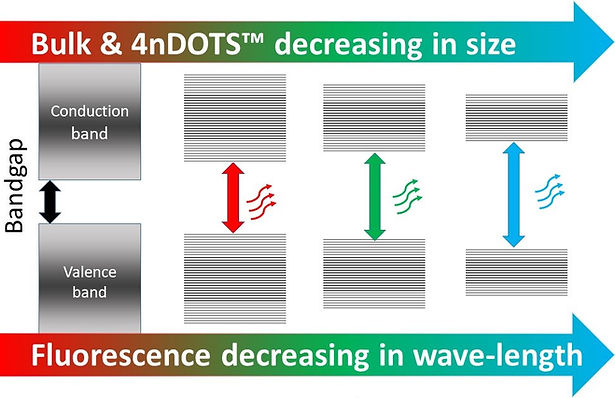
Figure 1: Splitting of energy levels in 4nDOTs™ due to the quantum confinement effect, band gap increases with decrease in size of the dot.
Fluorescent Nanoprobes Physicochemical Properties
Tunable Bandgap:
Due to their small size, the electrons in 4nDOTS™ are confined in a small space and the energy levels are quantized (Figure 1) [4, 5]. The discrete, quantized energy levels of 4nDots™ relate them more closely to atoms than bulk materials. As the size of the crystal decreases, the energy-gap between the highest valence band and the lowest conduction band increases, resulting in a color shift from red to blue in the emitted light. As a result, 4nDOTS™ can emit any color of light from the same material by changing their size. Because of the high level of control possible over the size of the nanocrystals produced, 4nDOTS™ can be tuned to emit any specific color of light [6]
​
Excitation and Emission Spectrum
4nDOTS™ show a broad excitation spectrum whereas the emission spectrum is narrow and separated from the peak of the excitation spectrum (typically > 100 nm), allowing easy separation between the two wavelengths. A single fixed wavelength light- or laser source in the UV/blue spectrum allows exciting a variety of different 4nDOTS™, each emitting in a different, distinct, defied and narrow wavelength (Figure 2a,b). As a consequence:
-
A lower cost, robust laser/light-source can be employed compared to the need of a tunable laser in the case of organic dyes.
-
Multiplexed imaging using a single light source can be used to excite multicolor 4nDOTS™ simultaneously without signal overlap. Their different narrow emission spectra can be distinguished, in contrast to the broad and thus overlapping emission spectra of organic dyes.
-
Emission spectra can easily be separated from the excitation spectrum, as they allow the excitation wavelength and the emission wavelength be separated by typically > 100 nm, in contrast to the case of organic dyes, where the excitation spectrum is narrow and often overlaps with the emission spectrum.
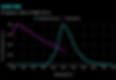

Figure 2a: Typical Excitation and Emission spectra of green emitting 4nDOT500
Figure 2b: Photoluminescence of 4nDOTS™. The materials emit different color of light by tuning the composition
Fluorescent Nanoprobes Surface Chemistry
​
Conjugated 4nDOTS™ provide precise binding specificity to biomolecules (Figure 3 below).

Figure 3: Schematic of a 4nDOT™: A: Core B: shell, C: Functionalizer, D: Agent for specific targeting (antibodies, primers, etc). 4nDOTS™ consist of some or all the elements shown above, depending on the costumer needs.
4nDOTS™ Applications
4nDOTS™ are particularly significant for optical applications owing to their bright, pure colors along with their ability to emit all colors of the VIS up to the NIR spectrum, coupled with their high efficiencies, substantially eliminated effect of photobleaching compared to organic dyes and high extinction coefficient. Examples include biomedical imaging, sensors, solid state lighting, displays and photovoltaics. [6, 7, 8]
The small hydrodynamic radius of 4nDOTS™ make them suitable for different bio-medical applications like biomedical imaging, biosensors, etc. At present, fluorescence based biosensors depend on organic dyes with a broad spectral width, which limits their effectiveness to a small number of colors and shorter lifetimes to tag the agents. 4nDOTS™ can emit in the whole spectrum, are brighter and substantially eliminate photo bleaching over time thus proving them superior to traditional organic dyes used in biomedical applications.[9]
Being zero dimensional, quantum dots have a sharper density of states than higher-dimensional structures. Their small size also means that electrons do not have to travel as far as with larger particles, thus electronic devices can operate faster. Examples of applications taking advantage of these unique electronic properties include transistors, photovoltaics, ultrafast all-optical switches and logic gates, and quantum computing, among many others. [10,11]
4nDOTS™ are fully biocompatible due to their aqueous medium and their controlled pH. This unique feature makes them ideally suited for use in in variety of bioimaging application (Figure 4) including:
-
ex vivo, in vitro long-term life cell imaging
-
in vivo animal targeting
In strong contrast, organic dyes require fixing the cells and thus do not allow time studies over a longer period with the same cell cultures as it is possible using bio-compatible 4nDOTS™

Figure 4: C6 glioblasioma cells, 4nDOTS™ - labeled. Incubation time 7 days. Fluorescence image obtained by singlephoton laser scanning confocal microscopy [12].
4nDOTS Order Information
PHORNANO offers a portfolio of fluorescent 4nDOTS with predefined emission wavelengths, as well as customized 4nDOTS on demand. An overview is provided in Table 1
​
Table 1: 4nDOTS emission wavelength, Item number, particle concentration and color of emission.
*) Emission wavelength can be customized between 490 nm (blue/green) and 620 nm (red). For emission wavelength of 580 nm (yellow), please ask for 4nDOT580. Other emission wavelengths are available upon request.
References:
​
1. Kastner, M. A. Physics Today, 1993, 46(1), 24.
2. Ashoori, R. C. Nature, 1996, 379(6564), 413.
3. Collier, C. P.; Vossmeyer, T.; Heath, J. R. Annual Review of Physical Chemistry, 1998, 49, 371.
4. Reimann, S. M.; Manninen, M. Reviews of Modern Physics, 2002, 74(4), 1283.
5. Bawendi, M. C.; Steigerwald, M. L.; Brus, L. E. Annual Review of Physical Chemistry, 1990, 41, 477.
6. Yoffe, A. D. Advances in Physics, 2001, 50(1), 1.
7. Nirmal, M.; Brus, L. Accounts of Chemical Research, 1999, 32(5), 407.
8. Sargent, E. H. Nature Photonics, 2012, 6(3), 133.
9. Wegner, K. D. Chem. Soc. Rev., 2015, 44, 4792-4834
10. Zhao, Y.; Burda, C. Energy & Environmental Science, 2012, 5(2), 5564.
11. Medintz, I. L.; Uyeda, H. T.; Goldman, E. R.; Mattoussi, H. Nature Materials, 2005, 4(6), 435.
12. Farias, P.M.A. Proc. SPIE 10481, Neural Imaging and Sensing 2018, 1048113